Words By Amy-Rose Collins
At the centre of our galaxy, there lies a heavyweight monster capable of devouring entire star systems in a single gulp. Three Laureates have the beast to thank for this year’s Nobel Prize in Physics. Roger Penrose, Reinhard Genzel and Andrea Ghez share the prestigious award, being credited with theoretically and observationally demonstrating the existence of our supermassive black hole, Sagittarius A*.
Black holes were once extremely heavy stars that exploded violently as supernovae at the end of their lives, only to succumb to gravity’s will and collapse to a single point in space. (Note that ‘supermassive’ refers to the sheer amount of matter – like stars and badly driven spaceships – that makes up a black hole, not its physical size.) With a gravitational field so intense, get too close to the centre, and nothing in the universe can travel fast enough to escape its pull – not even the light we need to see them. Thankfully, from our vantage point 26,000 lightyears away from Sagittarius A*, we’re not likely to be torn apart anytime soon.
Penrose received one half of the 2020 Prize for proving that Einstein’s mathematical theory of Relativity predicts the existence of black holes. His unique approach to the problem of collapsing material is widely regarded as the greatest contribution to the theory since Einstein. Penrose, who is also known for producing optical illusions such as the impossible triangle and Penrose steps, speaking to Nobel Media, recalled “[I started] thinking in a geometrical way, not really solving equations because you know it’s too complicated”.
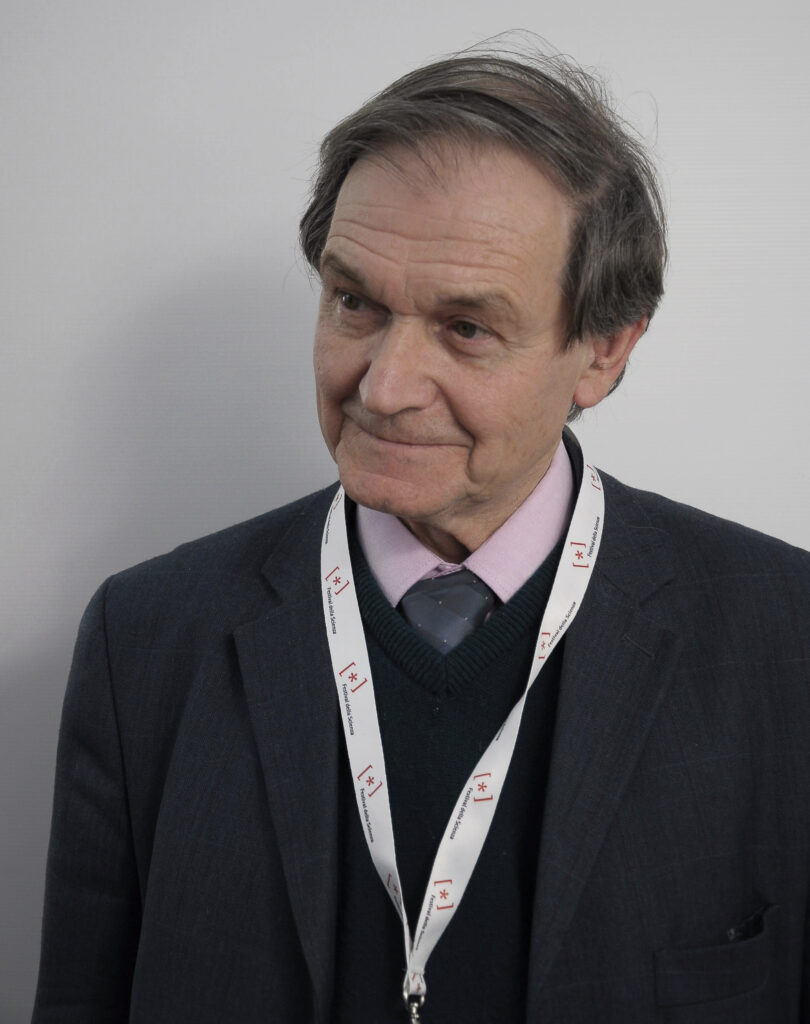
Prior to Penrose, Physicists were making assumptions about the symmetry of collapsing stars to simplify the equations they had to solve. In reality, bodies are rarely perfectly spherically symmetrical. The models’ simplicity, paired with calculations predicting a rather bizarre object, meant that many Physicists – including Einstein himself – did not believe that these solutions could be predicting the existence of black holes. However, in 1965, Penrose published a groundbreaking article describing his creation, ‘trapped surfaces’. He used these surfaces to argue that, even if the collapsing material is not spherical, once certain conditions are met, Relativity indeed predicts the collapse will produce a black hole.
Just years after Penrose’s success, scientists discovered that when matter falls into a black hole, energy is released in the form of incredibly bright electromagnetic radiation called a ‘quasar’. Using this newfound knowledge, an estimate of how common these quasars should be was made. The result indicated that most galaxies should have a black hole at their centre. In 1971, a paper arguing for the existence of a black hole at the centre of our own galaxy was published. It even gave us some ideas for how to find it, if it existed. If there is something with a strong gravitational influence relatively nearby, perhaps we could observe its influence.
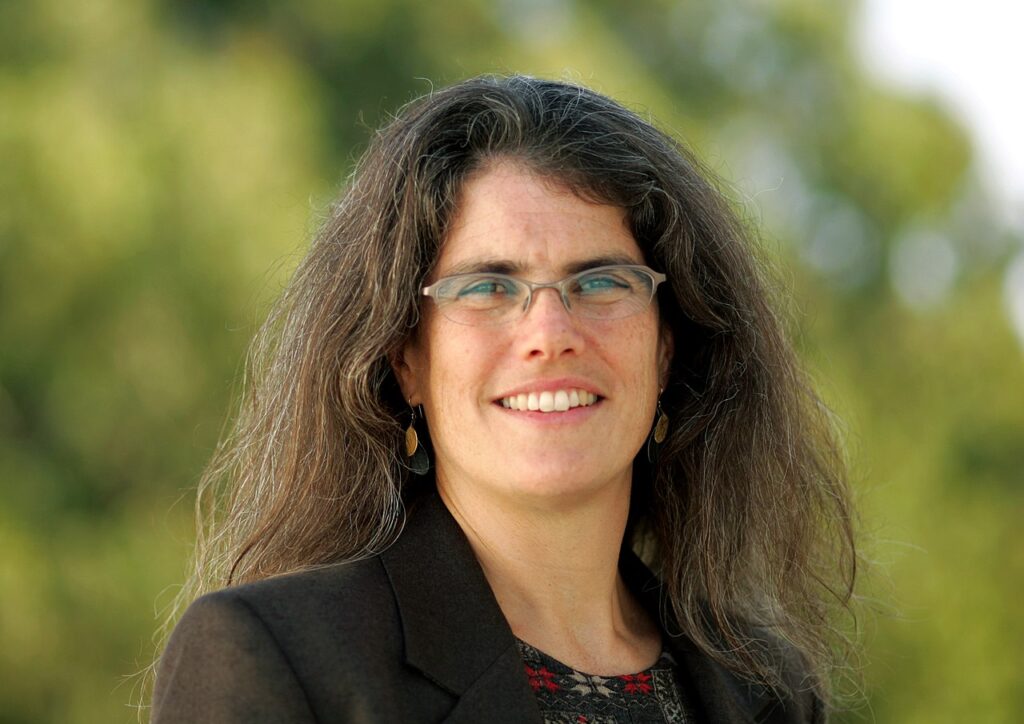
Genzel and Ghez share the other half of the Prize for the measurement and calculation of Sagittarius A*’s properties. Beginning in the 1990s, each led their own team of Astronomers in the search for evidence of a black hole in the centre of our own galaxy, the Milky Way. Our tiny homeworld is situated roughly two-thirds out from the centre of the flat, disk-like galaxy. This meant that Genzel and Ghez’s teams had to use large, near-infrared telescopes to ‘see’ through the billions of stars and clouds of dust in between us and their target. Their first challenge, however, was something far closer to home.
The atmosphere that surrounds our planet contains bubbles of gas that shift around each other depending on their relative temperatures, and can make far off objects appear to shift position. This is the same thing that happens around a candle flame, and is what makes stars appear to twinkle. To combat the blurring effects of the atmosphere on their measurements, Genzel and Ghez utilised newly available ‘adaptive optics’. In her 2009 TED talk, Ghez described the technology as “kind of like very fancy eyeglasses for your telescope”. The telescopes’ glasses consist of an additional mirror which is capable of rapidly changing shape in response to the guidance of a laser-created, artificial star. Since the actual position of the ‘guide star’ is known, the image received at the telescope can be used to measure where the atmosphere has ‘moved’ the guide star to, and the secondary mirror adjusted to correct for this unwanted distortion.
Atmosphere, dust, and unfathomable distance traversed in but a few decades, both teams began their clearest observations yet of the galaxy’s centre. But of course, what they were observing was not the black hole itself – for past a certain point, it gobbles up everything we could possibly use to see it – but the orbits of the closest thirty stars, which are all far enough away and moving fast enough to avoid certain doom. Genzel explains: “We are seeing not it, […], we are sensing its gravity”.
The star named ‘SO2’ proved vital in the teams’ calculations. It takes the star just 16 years to complete an orbit around the galactic centre (for comparison, it takes our solar system around 200 million years), which means that astronomers have had time to map its entire elliptical orbit. By using the data collected, the teams were able to reverse-engineer the properties of the object SO2 is orbiting. In papers published in 2009 and 2008 respectively, Genzel and Ghez’s results individually found that the object has a mass equivalent to around 4 million times the mass of our sun, and is squeezed into the same size as our solar system. The only currently known explanation is that the object must be a black hole.
At least, Physicists don’t have a better explanation yet. We know that Einstein’s Relativity can’t be applied in the centre of black holes, and there are many theoretical Physicists working on theories such as Quantum Gravity which may unlock the secrets that lurk there. After this peak into the heart of our galactic neighbourhood, how exciting to know that as our technology develops, we have a potential fountain of knowledge right on our doorstep.